Research
A lot of interesting research is going on in the Quantum Technologies group. On this page we highlight some of the exciting topics that we are working on. Also check out the page with recordings of a selection of our presentations.
Introduction

This optical micrograph captures many of the research themes of the Quantum Technologies group. The device is a controlled NOT circuits for linear-optics quantum computation. Here photons are used to encode and manipulate quantum information in an integrated-photonics platform. The chip - with many of these devices - is made using advanced nanofabrication techniques. For the detection of the single photons, superconducting single photon detectors are integrated on the same chip, and to program the quantum circuitry, optomechanical phase shifters are used. The latter are a spin-off of our other research themes: quantum optomechanics and optomechanics for science and technology.

Our research is organized around a number of central research themes: Quantum Optics, Quantum Optomechanics, Quantum Interfaces, and Optomechanics for Science & Technology. For each of these themes a subset of our core competences is used. Our expertise includes:
- Integrated optics & photonics
- Mechanical systems down to the nanoscale
- Advanced nanofabrication techniques
- Engineering and material science
- Cryogenics
- Vacuum technology
- Measurement automation and data processing
- Single photon sources
- Superconducting single-photon detectors
- High frequency measurements and low-noise electronics
- Feedback, noise, and control systems
- Modelling and simulations
- ...
For many of these competences, decidated equipment is needed. This can be high-end commercial products, but also home-build systems. More information can be found on our Equipment page.
With our themes and competences, we cover a wide range of topics in Quantum Technology, ranging from fundamental science all the way toward technology. Hence we are always interest in both academics and industrial collaborations.
Integrated quantum optics
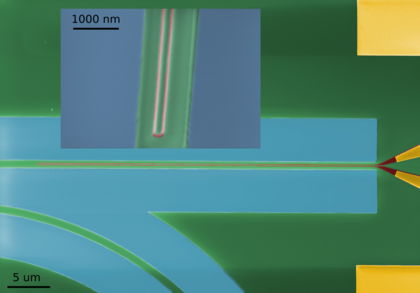

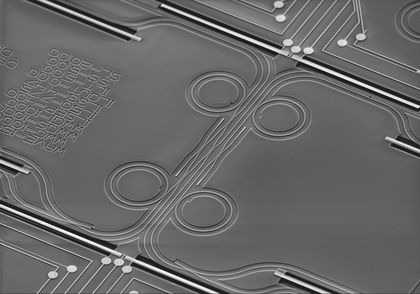
Many of the groundbreaking experiments in quantum optics were performed on optical tables full with carefully aligned lenses, mirrors, and waveplates. It is clear that this approach is not scalable to larger systems. For this, integrated optics is the way to go. We design, make, and measure chips with all the functionality to perform quantum optics on the chip. This includes source of single photons, quantum circuitry made of waveguides and directional couplers, and single photon detectors.
For the single photon sources we developed hybrid photonic circuits, where aluminum nitride - a material with a strong chi(2) nonlinearity - is grown on top of prefabricated silicon nitride circuits. This combines the controlled fabrication of low-loss SiN photonic circuits with the nonlinear optics capabilities of AlN. To learn more about this, you can watch our CLEO 2021 presentation here.

Optomechanics for Science and Technology
Optomechanics with 2d materials
2D materials such as graphene, WSe2, hBN can be made in freestanding single-atom thin membranes, making these ideal systems to study mechanics.
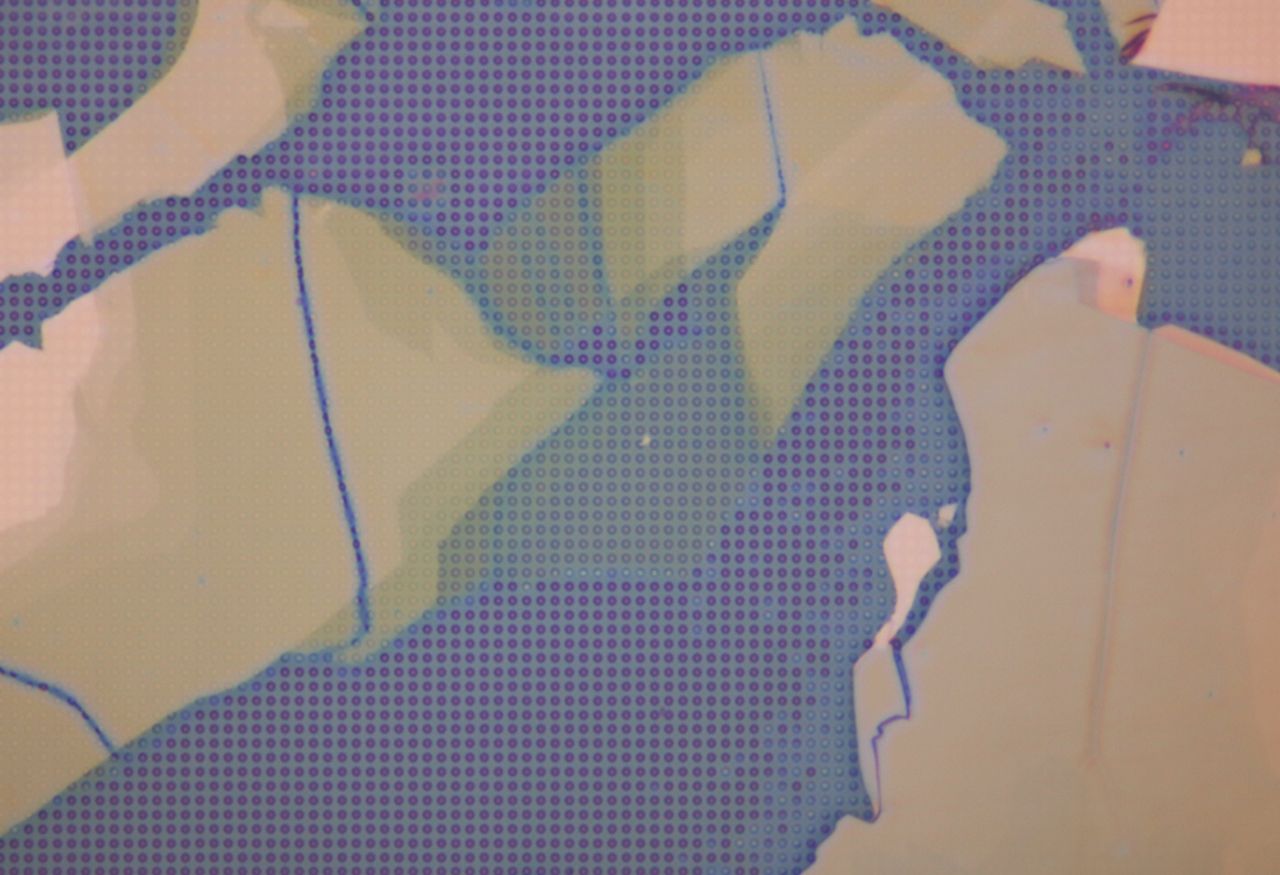
Quantum optomechanics
In our daily life, we encounter many different types of mechanical devices. These are all accurately described by the classical laws of motion. However, when making very small - nanomechanical - resonators and cooling them down to very low temperatures, quantum mechanics takes over. We are interested in studying the interplay between single photons and mechanical resonators, as well as using measurement-based techniques to manipulate the tiny vibrations of such systems, e.g. using feedback-assisted squeezing.
Quantum interfaces
Mechanical systems couple to almost anything. They can "feel" temperature, electric and magnetic fields, added mass, strain, and -importantly for optomechanics - also to light. By coupling a mechanical resonator to two different systems, an efficient interface can be created. This can be done between systems that would otherwise be very hard to couple directly, for example because the frequencies are many orders of magnitude different. The conversion can be either one way, or bidirectional. The holy grail is a device that works both ways without distrubing the signals, in particular without adding any noise. The ultimate limit in noise added by the interface occurs when the mechanical resonator is in its quantum ground state. We are interested in e.g. the conversion between microwave signals (as come e.g. from superconducting qubits) and optical wavelengths (as can be used for long distance quantum communication).
Some of the images shown on this page, originate from Prof. Poot's postdoctoral research in Prof. Hong Tang's group, Department of Electrical Engineering, Yale University.