Particle Collisions at High Energy
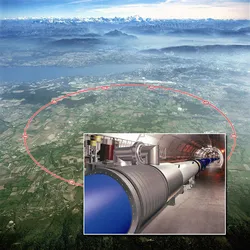
The controlled environments of high-energy particle colliders, such as the Large Hadron Collider (LHC), make them one of the main tools for testing the Standard Model of particle physics and searching for new particles and phenomena at the smallest distance scales. Indeed, during the last decades they have been the primary experiments in which new fundamental particles have been discovered and their properties measured. The most notable example is the discovery of the Higgs boson, the final ingredient of the Standard Model as we know it. However, the Standard Model is certainly not the final theory of Nature, most notably as it does not explain dark matter . The framework for describing particle interactions at the most fundamental level is based on relativistic quantum fields---the particles are their quantised fluctuations similar to the photons for the electromagentic fields.
To get the most out of the highly-accurate and precise data from the LHC, and possible future particle colliders such as an electron-positron collider, precise theoretical predictions are mandatory. Only by quantitative comparisons between the data and theoretical calculations, deviations from the known Standard Model can be found and information that might help in answering fundamental questions about nature can be deduced. Very broadly speaking, the T31 group focuses on developing and using precise predictions to understand nature at its smallest distance scales.
Remarkably, at the highest energies currently accessible to particle colliders all elementary particle interactions are weak, even the so-called strong interaction of quarks and gluons. This allows in principle for very precise calculations of the properties of particle collisions, requiring the inclusion of quantum fluctuations, which themselves can be used as tools to probe even smaller-distance phenomena than those expected from the energy of the colliding particles. Nevertheless, weak coupling at high energies does not guarantee that perturbation theory always works. When the process studied involves several distance scales, the quantum fluctuations are enhanced by the ratio of the different scales, and the straightfoward expansion in the small coupling breaks down, requiring what is called resummation. The group of Prof. Beneke focuses on developing methods to obtain accurate predictions even in this situation. In experiments, the collisions that allow to probe the shortest distances often involve hadrons, composite objects made of quarks and gluons whose size is of the order of the scale where the strong interaction is truly strong. To make predictions requires a careful separation of the short-distance hard scattering from the bound-state dynamics by a procedure called factorization. Understanding factorization of high-energy scattering beyond the leading order in the ratio of the scales has emerged as a new field, which plays a central role in the group's research. Specific topics:
- Development of effective quantum field theory methods in collider physics, especially beyond the leading power in the hard-scattering scale.
- Resummation techniques based on soft-collinear effective field theory (SCET), applications to the pair production of top quarks and other heavy particles.
- Implications of the fact that perturbation expansions in quantum field theory are not convergent series, but asymptotic, for particle physics phenomenology ("renormalons").
- Determination of the strong coupling, heavy quark masses
- Non-relativistic effective field theory
- Soft and collinear limit of the gravitational interaction (soft-collinear gravity)
You can find a list of publications from T31 in the INSPIRE HEP database
You can find information on master and bachelor thesis topics here