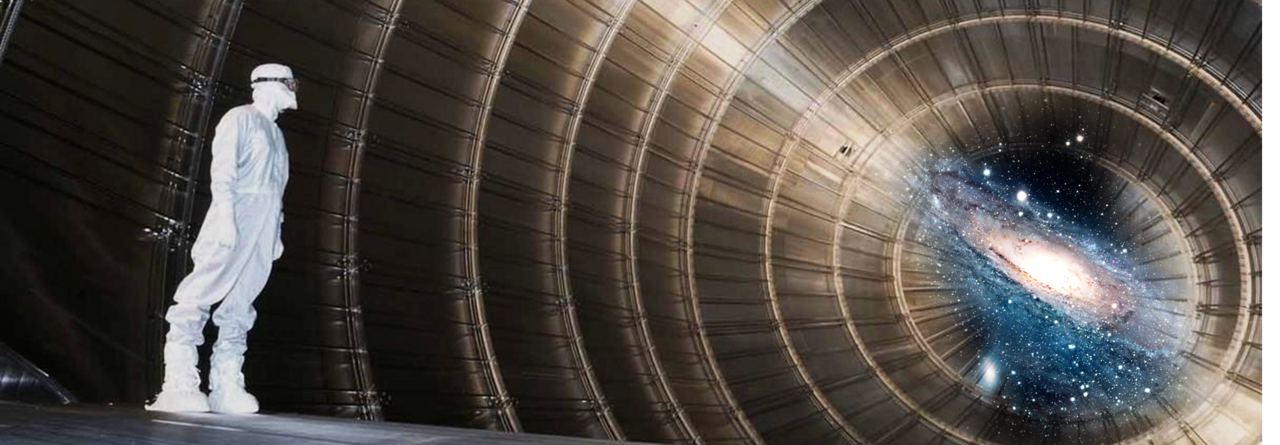
Our research is motivated by some of the most fundamental open questions in Astroparticle Physics: What is the neutrino mass and what is its origin? What is the reason for the matter anti-matter asymmetry of our universe? What is the nature of dark matter?
With a non-zero mass at least six orders of magnitudes smaller than the mass of electron the neutrino is an interesting misfit the Standard Model of Particle Physics. As the neutrino is the most abundant known matter partile in the universe, knowing its mass, would help to understand its role as cosmic architect of structures such as galaxies and galaxy cluster. With the large-scale KATRIN experiment we are performing a direct measurement of the absolute neutrino mass with unprecedented sensitivity.
Another unique property of the neutrino is the possibility that it may be its own antiparticle. This feature would imply that processes can occur in in which matter is created, without a balancing amount of anti-matter. This, in turn, is a prerequisit to explain one of the biggest mysteries, the matter anti-matter asymmetry of our univere. With the next-generation LEGEND experiment we are searching for an as-of-today undiscovered reaction, the so-called neutrino-less double beta decay, which would prove that neutrinos are their own anti-particles.
One of the most compelling open questions in physics today is the nature of dark matter. From indirect observations we know that dark matter makes up 25% of the energy content of our universe and more than 80% of the matter of our own galaxy. Nevertheless, it remains elusive what this form of matter is made of. Two promising candidates for dark matter are a new form of neutrinos, so-called sterile neutrinos with a mass in the kilo-electron-volt regime and axions, which are prediced in the theory of Quantum Chromodynamics.
The KATRIN experiment, upgraded with a new Silicon-Drift-Detector focal plane detector, TRISTAN, will enable a search for sterile neutrinos with an improved sensitivity. The qualities of the TRISTAN detector, such as its good energy resolution and low threshold, make it ideally suited for IAXO, an experiment, which searches for axions emitted from the sun. As TRISTAN likes to explore the unknown, we also allow him to take part in a cube satellite space mission, ComPol, where he will observe X-rays from a black-hole binary system.
KATRIN
The goal of the Karlsruhe Tritium Neutrino experiment (KATRIN) is to determine the absolute neutrino mass. To do so, KATRIN performs an ultra-precise measurement of the tritium beta-decay spectrum close to its endpoint, where the neutrino mass leads to a small distortion of the spectrum. KATRIN consists of a 70-m long beamline, combining a high-luminosity tritum source with an ultra-precise spectrometer. As of today, KATRIN provides the strongest limit on the neutrino mass of m < 0.8 eV, based on this model-independent measurement technique. Over the next years we aim to reach a sensititiy close to 0.2 eV. KATRIN is situated at the Karlsruhe Institute of Technology and an international research collaboration comprising around 150 members from 17 institutes in six different countries is involved in this experiment. Our Research Group concentrates on the data analysis and on developing a novel detector system for KATRIN, known as TRISTAN.
TRISTAN
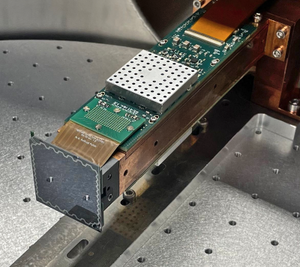
Owing to its excellent source and spectrometer properties, the KATRIN experiment enables us to not only determine the neutrino mass, but also to search for a new variant of the particle: The so-called sterile neutrino. Unlike the known active neutrinos, this hypothetical particles interacts even weaker, and can have any mass. A sterile neutrino with a mass in the keV region is a viable dark matter candidate.
A sterile neutrino with a mass in the keV range can leave a characteristic signature in the tritium beta decay spectrum. As KATRIN operates one of the strongst tritium sources in the world for scientifc reasearch, it is the ideal place to conduct a search for sterile neutrinos. The KATRIN beamline, however, is designed to precisely measure only the region close to the endpoint of the spectrum, where we expect the signature of the neutrino mass. In order to search for keV-scale sterile neutrinos the entire tritium spectrum must be detected. This extension leads to multiple challenges, for example the rate of beta-electrons will be many orders of magnitudes higher. This is why we develop a novel detector system, TRISTAN. Is consistes of more than 1000 Silicon Drift Detector pixels, over which the high flux of electrons will be distributed. Each pixel can measure precisely the energy of each electron.
We have finalized the design of the TRISTAN detector and we built a complete TRISTAN module, which comprises 166 SDD pixels (see photograph). In 2026 nine TRISTAN modules will be installed in the KATRIN beamline.
LEGEND
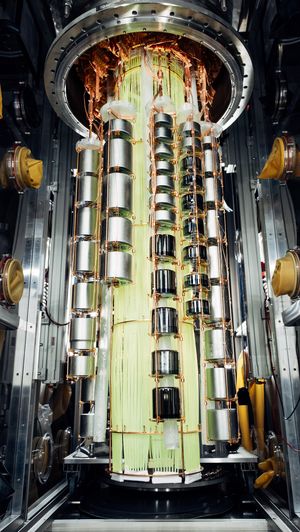
The Large Enriched Germanium Experiment for Neutrinoless double-beta Decay (LEGEND) is a next-generation 76Ge-based neutrinoless ββ (0υββ) decay experimental program. The ultimative goal is to operate one ton of high-puirty Germanium detector (HPGe) enriched in the isotope 76Ge in ultra-clean conditions and probe the 0υββ-decay of 76Ge at half-lifes longer than 1028 years.
The first stage of the experiment (LEGEND200) is currently in progress at the Gran Sasso National Laboratory (Laboratori Nazionali del Gran Sasso) in central Italy. LEGEND200 operates 200 kg of enriched HPGe detectors at cryogenic temperatures of 87K in a cryostat containing approximately 60 tons of liquid Argon. The experiment will take data for approximately 5 years and probe the 0υββ-decay of 76Ge at half-lifes of 1027 years.
The next stage of the experiment (LEGEND1000) aims to operate 1000 kg of enriched HPGe detectors and is currently in the research & development (R&D) stage. Once operational, the experiment will take data for approximately 10 years and probe the 0υββ-decay of 76Ge at half-lifes of 1028 years.
The main experimental challenge is to achieve an ultra-low radioactive background. At half-lifes of 1028 years, only around one 0υββ-decay of 76Ge is expected per year. In order identify such a small signal in the presence of naturally occuring radioactive decays, very sophisticated techniques have to be employed. This ranges from the use of extremely clean materials to advanced data analysis methods.
Our research team at TUM is involved both in LEGEND200 and LEGEND1000, specialising in data analysis and the development and characterisation of ultra-clean readout electroncis for HPGe detectors.
IAXO
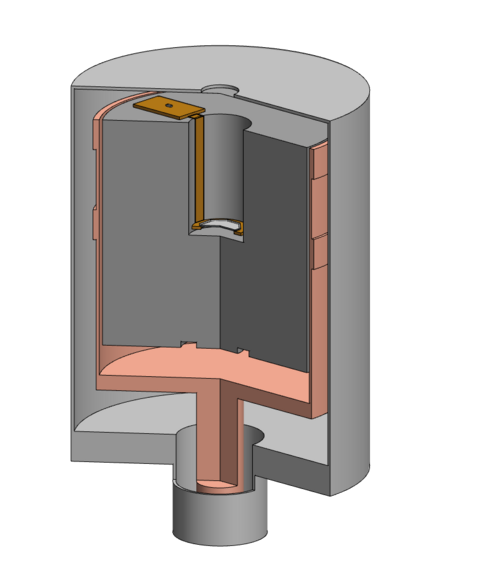
The International Axion Observatory (IAXO) aims to detect solar axions as they are back-converted into X-rays along a strong magnet pointing towards the sun. X-ray optics will concentrate the axion signal onto cm-scale ultra-low background detectors. This detection could single-handedly resolve shortcomings in our description of strong interactions and represent the first observation of a dark matter candidate in a laboratory setting.
As such axion signals will be rare, highly efficient X-ray detectors with a background performance similar to deep-underground experiments are required. Silicon drift detectors (SDDs) feature unique properties for this task. No structural components towards the telescope and only a very thin dead-layer allow for maximal X-ray absorption efficiency at solar axion energies. Construction from semiconductor grade materials and great freedom for sophisticated shielding strategies indicate great potential for ultra-low backgrounds.
BabyIAXO, the first stage of the IAXO program, is currently under construction at Deutsches Elektronen-Synchrotron (DESY) in Hamburg. It will represent a testing ground for IAXO technologies, while probing unexplored axion parameter space. We aim to deploy a passively shielded SDD detector setup for this measurement. For the even more challenging IAXO phase, we are developing a novel all-semiconductor active-shield X-ray detector, which consists of a single-pixel SDD operated inside a large-volume well-type high-purity germanium (HPGe) detector. This SDD-in-HPGe detector aims to acchive ultimate low-background performance for above-ground X-ray detection and boost the IAXO sensitivity to solar axions.
ComPol
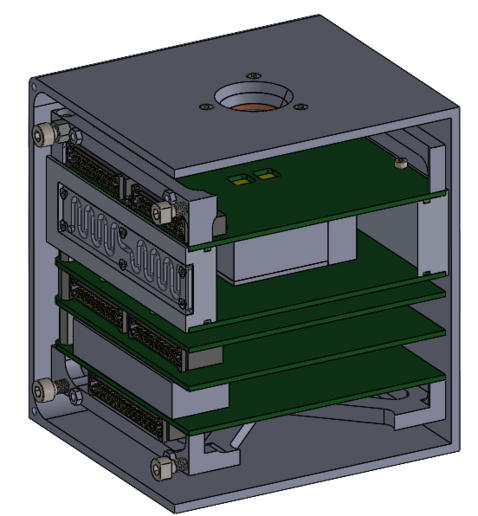
ComPol is a CubeSat mission aiming at measuring the X-ray polarization from the black hole binary (BHB) Cygnus X-1. The satellite will have a size of 3 CubeSat units (approx. 10x10x34 cm3) and it will fly in a low Earth orbit (~500km altitude) taking data for minimum one year.
The scientific motivation for the project is to futher understand BHBs, e.g. their geometric structure or accretion dynamics. Measuring the hard X-ray polarization makes it possible to distinguish between the two main mechanisms for the X-ray production. One option is the production by inverse Compton scattering in a hot gas cloud around the black hole. This process yields unpolarized emission while the second option, the X-ray production from synchrotron radiation, would cause a non zero degree of polarization, polarized in the plane of motion.
The used detector system is composed of two layers, a Silicon Drift Detector (SDD) on top of a CeBr3 calorimeter. The latter is a scintillator read out by a Silicon photomultiplier matrix. The intended X-ray interaction is forward Compton scattering in the SDD and subsequent absorption in the CeBr3 crystal. The selected detector combination makes it possible to measure the full kinematic of Compton scattering down to a few tens of keV and up to a few hundred keV. The characteristic relation for Compton scattering between the initial energy, the scatter angle, and the deposited energy allows for an event-wise reconstruction. Together with the intrinsic dependence of the azimuthal scatter angle on the initial polarization vector one can determine the degree of polarization and the polarization plane of the incoming radiation.